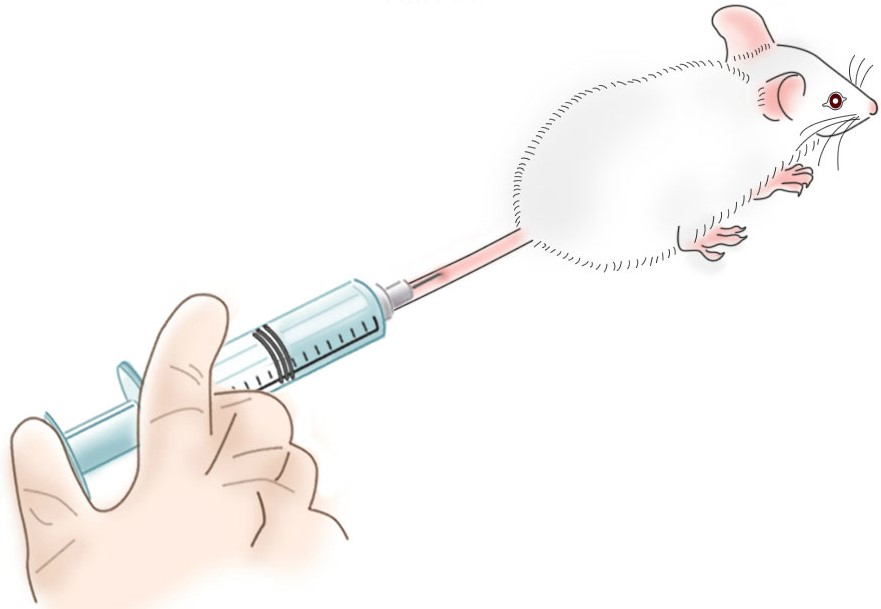
Systemic Administration of Clodronate Liposomes via Intravenous Injection
We are continuing to review publications related to intravenously injected clodronate liposomes. This discussion will be updated with any pertinent information as we find it or whenever new information is published.
The intravenous route of administration is used in almost half of the published studies on liposomal clodronate. Using intravenous injection of clodronate liposomes limits their access to the liver, spleen and bone marrow along with circulating monocytes. Clodronate liposomes have access to the phagocytic cells of these tissues through specialized vasculature known as sinusoids but cannot otherwise leave the vascular system in normal, healthy animals. The liver and splenic sinusoids possess a discontinuous basement membrane which allow not only liposomes, but also pathogens, large proteins and cells to exit the circulation, however the bone marrow sinusoids have no basement membrane. Endothelial cells lining the bone marrow sinusoids are supported by the constituent cells of the bone marrow such that when the bone marrow is depleted by radiation or chemotherapy, the sinusoids collapse and reappear only when the bone marrow is regenerated.
Small liposomes (<200 nm) can escape the vasculature in areas where inflammatory mediators induce the endothelium to allow extravasation of larger proteins and inflammatory cells. Monocytes can act as “trojan horses” in delivering drugs that have been released from previously phagocytosed liposomes by leaving the vasculature and maturing into tissue macrophages as is their normal fate, or the migration may be in response to inflammation. This is not usually a mechanism for clodronate delivery by clodronate liposomes except when they are locally administered in certain tissues such as the CNS, intrapulmonary space or possibly bone marrow where influx/efflux of water-soluble molecules is limited.
Intravenous injection of clodronate liposomes in rodents is typically accomplished through the lateral tail vein, but retro-orbital intravenous access is considered by some to be a superior method for intravenous delivery. Regardless of the access site, intravenously injected liposomes initially pass through the lung capillary beds before being circulated throughout the rest of the body from the aorta. The liposomes continue to circulate until removed by phagocytic cells of the tissues mentioned above. Familiarity and consideration of the circulation route of liposomes will allow scientists to better understand and utilize liposomal clodronate in their studies. Using the proper dosing technique along with appropriate storage and handling of Clodrosome® as described below will ensure the maximum depletion results with no adverse effects in treated animals. Clodrosome® is not inherently toxic to animals when used as directed. Unexpected deaths cannot be attributed to Clodrosome®; improper dosing methods and opportunistic infections are two of the major causes of unexplained deaths in Clodrosome®-treated animals.
Enhanced monocyte margination due to clodronate liposome administration or as a result of some pathogenic or inflammatory models must be considered when evaluating monocyte depletion as well as when monitoring blood levels of cytokines. Marginated monocytes are known to release higher levels of certain cytokines into the bloodstream, therefore the correlation between circulating monocyte counts and cytokine levels may not be accurate. When liposomes or other particles are injected into animals at sufficient doses, the phagocytic cells of the reticuloendothelial system (RES) become saturated and unable to take up more particles until their current load is digested or otherwise sequestered. This state, known as RES blockade, may be used as a method of enhancing the circulation time of liposomes or it may explain differences in the effects/biodistribution of liposomes after multiple injections. If clodronate liposomes are being used for complete systemic depletion of macrophages, the rate of repopulation of the phagocytes within the various organs and blood must be taken into account, especially considering that monocyte counts begin to increase between 24-30 hours in mice while liver and splenic macrophages may remain depleted for up to a week or more. During repopulation, the relative levels of various subpopulations of phagocytes can be considerably different from steady state distributions.
Depletion of macrophages via intravenous administration of clodronate liposomes is usually a “means to an end” rather than the focus of most published studies, however unless the behavior of the liposomes and the depletion/repopulation pattern in the chosen model in the chosen species has been well characterized in previous studies, data may need to be collected during the course of the study. Consideration of the appropriate depletion controls and limiting “assumptions” will allow for unambiguous interpretations of the resulting data. The most common method of dosing clodronate liposomes is by intravenous injection; about half of all clodronate liposome studies published thus far use the intravenous administration route. Direct administration into the bloodstream ensures that all phagocytes having direct access to the blood can potentially engulf clodronate liposomes. However, just as with all liposomal drug delivery, the liver and spleen are so highly efficient in removing foreign particles from the bloodstream, the exposure of phagocytes outside of these organs to blood-borne clodronate liposomes can be limited.
Unmodified, micron-sized clodronate liposomes will be quickly removed from the bloodstream, usually in less than 1 hour. Having said this, liposomes cannot leave the bloodstream through veins and arteries. In normal animals, the only areas where liposomes can interact with cells outside the vascular system is in the sinusoidal capillaries of the spleen, liver and bone marrow [1]; sinusoidal capillaries are a type of fenestrated capillaries (containing small holes or fenestrae) with a very loose structure, little basement membrane and large fenestrae which allow equally large molecules, cells and pathogens to pass through their walls.
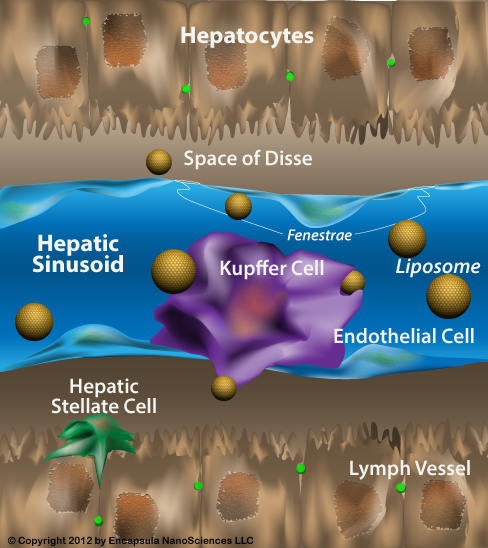
Bone marrow and its sinusoids comprise a unique environment. The bone marrow (BM) was also thought to be composed of sinusoidal capillaries located within the marrow sinus similarly to other components of the mononuclear phagocyte system (MPS, aka RES, or reticuloendothelial system), but the size of these fenestrae have been reported to be only 85-150 nm in mice and therefore only accessible to liposomes in the ≤120 nm range. The predominant mode of larger liposome uptake by bone marrow appeared to be by the perisinal macrophages which extend pseudopodia between the endothelial cells and into the marrow sinus [2, 3, 4] in order to capture blood-borne particles, including damaged RBC, although this mechanism has only been directly observed in rabbits and marmosets. However, given that larger liposomes do indeed enter the bone marrow in rats and mice, we assumed that all bone marrow sinusoids have some mechanism by which macrophages come into contact with intravenous liposomes sized in the micron range. These authors also warn that there appears to be a large disparity between species when looking at the mechanisms of bone marrow uptake with mice and rats prone to limited marrow uptake of liposomes when compared to rabbits and larger mammals.
This rather confusing description of bone marrow sinusoids was clarified by Kopp, et al. who describe BM sinusoids as having no basement membrane unlike other vascular components [17]. They describe marrow sinusoids as being lined by specialized bone marrow endothelial cells (BMEC) which are only supported by the cells of the bone marrow including hematopoietic stem cells and progenitor cells. Furthermore, unlike other vascular tissues in which the vascular endothelial cells are supported by a collagenous basement membrane, the BM sinusoids disappear when the cells of the BM are destroyed by radiation or certain chemotherapies as there is no other scaffolding to support the BMEC forming the sinusoids. The sinusoids are reconstructed as the BM is replenished. Therefore, access to the BM is dependent upon BMEC adhesion molecules (P-selectin, E-selectin, VCAM-1) and the various signalling events which induce the BMEC to separate from each other thus allowing access to the BM. The absence of the basement membrane suggests that there is no size restriction in the uptake of blood-borne particles into the BM.
More recently a bone marrow macrophage subtype, known as the osteomac, has been reported susceptible to intravenous clodronate liposome depletion by the retro-orbial route [9]. Osteomacs and other bone marrow macrophages (CD169+) have been shown by at least 2 groups to be key players in controlling the release of hematopoietic stem cells (HSC) into the circulation [13, 14] leading to the suggestion that intravenously delivered clodronate liposomes may be used to increase stem cell recovery in the blood post-treatment by granulocyte colony stimulating factor (G-CSF). G-CSF is used to stimulate production of hematopoietic stem and progenitor cells for collection prior to transplantation.
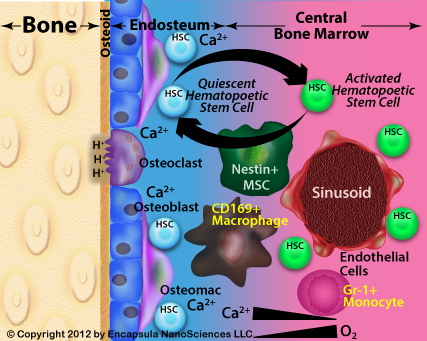
Application of fluorescent labeled liposomes to this model should shed light on precisely which populations of bone marrow macrophages phagocytose liposomes, where within the niche the uptake occurs and ultimately, whether or not osteomac depletion by clodronate liposomes plays a direct role in HSC mobilization. This is an example of how important it is to carefully consider the possible, or most likely, areas where liposomes can and cannot leave the vasculature as well as what happens to them when they have the opportunity to interact with extravascular tissues. For the most part, any tissue interaction is limited to phagocytic uptake since liposomes (including so-called “stealth” liposomes) have not been shown to migrate from the immediate perivascular region although their contents can be transported by migrating macrophages. It is also imperative to no longer consider a liposome, which has been phagocytosed, to be a liposome. Once internalized, liposomes are destined to be digested by the phagocyte, in which case the encapsulated cargo may be delivered by the macrophage as discussed later on this page. Even liposomes designed to be resistant to lysosomal degradation appear to remain inside a terminal endosome. We are not aware of any plausible reports of phagocytosed liposomes “reappearing” as an intact liposome as some seem to suggest.
Another point which we constantly reiterate is not a “given”, is the assumption that unencapsulated or free clodronate is not toxic or otherwise has no effect on cells in general. Free clodronate has been shown to be toxic to macrophages in vitro, albeit at higher concentrations than required for liposomal clodronate toxicity and elicit morphological changes in pulmonary endothelial cells in vivo (see discussion on the Intrapulmonary administration page). In fact, the only cells with demonstrated susceptibility to intravenously injected free clodronate are osteoclasts. The authors investigating bone marrow phagocyte depletion with clodronate liposomes above assume that free clodronate released from dying macrophages within the BM has no effect on the surrounding cells, however given that the bone marrow constituents, especially those located toward the endosteal region (remote from the blood flow in the sinusoid), experience a much more limited exchange rate of extracellular molecules, including oxygen, it is conceivable that clodronate release from dead/dying macrophages could result in a substantial concentration of free clodronate in the region. While we cannot cite any specific data in these studies that would indicate a substantial contribution of free clodronate, we believe that the presence of free clodronate in the BM is a distinct possibility and that the effects of liposomal and free clodronate on the various cell types in the bone marrow should be investigated. For example, Kellinsalmi, et al. have demonstrated clodronate-induced inhibition of calcium deposition by human MSC-derived osteoblasts at concentrations as low as 1 nM [20]. Bone marrow targeting of liposomes has been reported using a unique liposome formulation containing carboxylic acid based lipids, which display succinate on the surface of the liposome, as the targeting moiety [2] although this targeting mechanism has not been applied to clodronate liposomes to date.
Sites of Inflammation Allow Smaller Liposomes to Escape the Vasculature. If any site(s) of inflammation are present in the animal, inflammatory mediators cause vascular endothelial cells to separate (where they are normally closely associated by tight junctions) forming openings in the capillaries as large as 400 nm. Therefore, liposomes that are smaller than these openings can escape the vasculature in areas of inflammation including solid tumors, focal infections, tissue injury, etc. A similar situation exists in areas of angiogenesis (new capillary growth) commonly found in solid tumors and damaged tissue where inflammation will also be a factor. This is the basis of passive targeting of smaller liposomes, however for most applications of clodronate liposomes, micron-sized clodronate liposomes only leave the vasculature through the spleen, liver and bone marrow sinusoids where they are predominantly phagocytosed by specialized macrophages (Kupffer cells in the liver, marginal zone macrophages of the spleen, and perisinusoidal macrophages of the bone marrow).
Monocytes and Macrophages Can Deliver Liposomal Contents, but Not Liposomes. Another mechanism by which liposomal contents can be delivered to tissues is often known as the trojan horse mechanism. Monocytes that have phagocytosed liposomes in the bloodstream, then migrate into tissues for maturation into tissue macrophages serve as the trojan horses. Of course, this mechanism is dependent upon the active liposomal drug being able to escape the monocyte/macrophage into surrounding tissue, or in the case of many pathogens, the monocyte/macrophage phagocytosing the pathogen which is then killed by the liposomal drug previously taken up by the monocytes from the bloodstream. This mechanism has been reported as responsible for delivering serotonin across the blood-brain barrier [11]. Clodronate liposomes can be used to verify the trojan horse mechanism by demonstrating that the drug or other compound is not delivered to the extravascular tissue in the absence of monocytes which have been depleted by clodronate liposomes.
Scientists also question the possibility of clodronate delivery by monocytes/macrophages (especially dying cells). In most cases where the clodronate liposomes have been dosed systemically (i.v. or i.p.), free clodronate released either from the liposomes or dying phagocytes is quickly diluted and excreted so that other cells are not exposed to free clodronate. Additionally, free clodronate cannot access the cytosol of most cells and in cases where it has been delivered intracellularly to cells other than mononuclear phagocytes (i.e. neutrophils, eosinophils), it doesn’t appear to be toxic to those other cell types [12]. However, in the case of many local delivery methods for clodronate liposomes, released clodronate may not be quickly diluted or otherwise removed from the site (see detailed discussion on the Intrapulmonary administration page), in which case the effect of free clodronate must be investigated with the appropriate controls. As discussed above, the bone marrow may be another location in which clodronate may accumulate when released from dead/dying macrophages, however this hypothesis has not been proven nor disproven to date.
Free clodronate controls should always be considered and/or investigated in vitro since the released clodronate may achieve substantial concentrations because the incubation conditions provide a limited dilution and the clodronate has no avenue for removal from the medium. While liposomal clodronate usually demonstrates an IC50 (concentration required to kill 50% of the cells or limit the growth of cells by 50%) of an order of magnitude higher than free clodronate, the experimental protocol should take into account the possibility of clodronate release from liposomes or dead/dying cells reaching a concentration that will affect the cultured cells.
Alternative Sites of Intravenous Injections
Many investigators only consider the lateral tail vein as an injection site for intravenous liposome dosing and it is the most common route used in rodents. However, some advocate intravenous injection via the retro-orbital sinus (abbreviated RO) as more humane, less traumatic and easier to master [5] than tail-vein injections, while other institutional animal use and care committees require additional justification for utilizing this route of intravenous administration. Of course, many animals do not have tails that enclose large, accessible veins. Rabbits are usually injected through a marginal ear vein, guinea pigs and hamsters through the penile vein and dogs through the cephalic or saphenous veins, although many species can be injected retro-orbitally; proponents suggest that the negative visual image of an animal being injected “in the eye” is the primary reason that this technique is not more commonly used. The method is preferred by some for small animal radionuclide studies (preclinical studies, in particular) as faster and less prone to accidental dissemination of radioactive compounds [6, 7]. The technique appears to be superior to tail-vein injections (or other venous routes) for neonatal mice and C57BL/6, or other dark-colored mice, for which the lateral tail veins are more difficult to visualize. The same dose-volume considerations apply to RO injections as tail-vein injections and the same dose volume is used regardless of the injection site. Retro-orbital intravenous delivery of clodronate liposomes has been used in several studies including those performed on hamsters [8] and mice [9]. Studies comparing retro-orbital and lateral tail vein injections conclude that there are no detectable differences in the delivery of most compounds dosed by the two methods.
From the Liposome’s Point of View
Consideration of the circulation route of liposomes (shown here) post-injection may decrease the gap in expectations vs. results when dosing liposomes. This diagram will also verify our previous statement that there is effectively no difference in the liposome circulation route dependent upon the intravenous injection site, reiterating that liposomes cannot leave the circulation through larger veins or arteries. After injecting liposomes into any venous site, the first capillary bed that they encounter is in the lungs from the right ventricle of the heart. Therefore, if the liposomes are aggregated or too large to pass through the lung capillaries, the animal will show signs of respiratory distress immediately after injection of the liposomes; avoiding respiratory distress when dosing clodronate liposomes intravenously is discussed in detail later on this page.
After passing through the lung capillary bed where the CO2-O2 exchange takes place, the liposomes continue into the left atrium, then left ventricle, of the heart and are distributed throughout the body from the aorta. A portion of the liposome dose will distribute into all arteries throughout the body and pass through the various organ capillary beds prior to re-entering the venous circulation. As discussed above, in a normal, healthy animal, clodronate liposomes will not leave the vasculature except in the spleen, liver or bone marrow. Thus, the vast majority of the liposome dose which enters the subclavian and jugular arteries and circulates through the upper portion of the body will return to the right heart. Only a very small portion of these liposomes will be taken up by the bone marrow in the upper torso. Likewise, most liposomes which enter into the renal and iliac arteries will return to the right heart where these liposomes will begin their second pass through the body with a slightly larger number of liposomes being taken up by the larger bones’ marrow in the lower body than in the upper body. The majority of the clodronate liposome dose which enter the mesenteric and celiac arteries will be retained in the spleen and liver. While we have been unable, thus far, to locate specific information on the blood flow distribution in rodents and other common lab species (i.e. what proportion of the blood leaving the aorta enters the mesenteric and coeliac arteries and is filtered through the liver during the first pass?) most of the clodronate liposomes will be filtered out by the spleen, liver and bone marrow within the first few passes. Of course, if these were 100 nm liposomes and included PEG on the surface, some would continue to circulate for a couple of days or more. However, clodronate liposomes are designed to leave the circulation quickly through phagocytosis.
While we have generally referred to the entire liposome dose and all of the liposomes, it is important to note that some of these liposomes will be destroyed upon entering the bloodstream. This means that if we were to attempt to identify the location of the entire injected clodronate liposome dose at any given time, we could never recover 100% of the injected dose (even outside of experimental error) because a certain portion (dependent upon the composition and morphology) of the liposomes will succumb to disruption by various serum proteins. This phenomenon is not usually of any consequence when dosing clodronate liposomes, but it is a factor often excluded when considering the fate of intravenously administered liposomes.
Intravenous Injection Precautions
Clodrosome® is usually dosed intravenously to mice and rats in a relatively large volume, or about 1% of their body weight. This, along with the fact that the Clodrosome® suspension is slightly more viscous than water, increases the possibility of adverse reactions due to improper injection techniques. When injecting Clodrosome® intravenously, the following guidelines will limit the potential for adverse reactions, including death. If the technician performing the injections is unfamiliar with injecting larger volumes (≥1% of the animals body weight) and/or viscous suspensions, it is useful for the technician to practice on non-experimental animals prior to initiation of the experimental protocol. Extra animals should be on hand should animals demonstrate suspicious behavior during or after Clodrosome® treatment.
The precautions listed below apply to any intravenous injection method or site since the liposomes will always initially pass through the lung capillary bed before they enter the arterial circulation for distribution throughout the animal. Prior to its expiration date, uncontaminated Clodrosome® (which has been maintained according to package instructions) is not toxic when dosed intravenously to animals according to the following guidelines. Animals expiring during the experimental protocol for reasons unrelated to the model should be necropsied and examined for opportunistic infections or other causes of premature death. Premature deaths, outside of injection-related adverse reactions, cannot be attributed to Clodrosome® treatment alone.
- Plan the experimental setup and protocol keeping in mind that the animals receiving Clodrosome® will become immune-suppressed within hours after treatment making sterile procedures for dosing and handling the animals more important than usual.
- While full-blown immunocompromised animal containment is usually not necessary (depending on the size and potential for contamination in the facility), extra precautions need to be implemented, such as more frequently supplying clean bedding, water-bottles and food on a daily basis, as well as closely monitoring the animals for signs of infection or illness not related to the experimental model.
- Take into account that Clodrosome®-treated animals may succumb to infectious disease models at a higher rate than normal animals requiring shorter intervals for assessing the animals’ conditions.
- A significant number of unexpected deaths, outside of injection-related adverse reactions, should be investigated as it is likely due to opportunistic infections whose source must be eliminated from the facility environment or handling procedures.
- Inflammation or necrosis at the injection site of only a few animals suggests contamination during the injection procedure while such reactions in several animals can be an indication that the clodronate liposome suspension has been contaminated. In this case the validity of the experiment should be seriously questioned.
- Generally, the maximum tolerable volume (ml) dosed to animals in a single, intravenous bolus should be limited to approximately 1% of the animal’s body weight in grams.
- For example, the maximum volume dosed to a 20 g mouse is 0.2 ml. Many sources will cite more conservative guidelines of 5 ml/Kg (0.1 ml/20 g mouse) or a maximum 4-5% dilution of the total blood volume (TBV~1.2 ml/20 g mouse X 5% = 0.06 ml/mouse), however, if the technician is experienced in maintaining a slow, steady injection rate along with the other precautions listed here, the larger volume is well tolerated.
- In the event that the investigator suspects that injection-related adverse events are interfering with the study, Clodrosome® can be diluted and dosed via intravenous infusion or smaller doses may be evaluated for effectiveness. However, this is rarely necessary.
- Clodrosome® should be at RT for dosing to healthy animals; infected or otherwise compromised animals (i.e. SCID or nude mice) may require Clodrosome® be warmed to the animal’s body temp prior to injection.
- Animals that are dosed intravenously with larger volumes of cold fluids can go into hypothermic shock.
- Note that Clodrosome® should be maintained at 4°C as much as possible. Consider making a sterile transfer of the total volume required for a single experiment from the original vial to another sterile container which can be warmed to the appropriate temperature, if the animal dosing protocol is expected to require Clodrosome® to be maintained at elevated temperature for longer than an hour.
- Immediately before extracting Clodrosome® from the vial, visually inspect the suspension, making sure that it is homogeneous with no visible particulates.
- Occasionally, liposomes will “settle” toward the bottom of the vial in which case the vial should be slowly and smoothly inverted several times.
- Never vigorously shake liposomes; they will not “break”, but lipid-stabilized air bubbles can be introduced into the suspension. If these are transferred into the syringe and dosed to an animal, it could be seriously injured or killed by air emboli (air bubbles introduced into the bloodstream).
- Also visually inspect Clodrosome® once it is drawn into the syringe to ensure that no air bubbles have been introduced during its extraction from the vial. Following proper syringe-filling and air-bubble-removal procedures should prevent any incidents due to air emboli.
- Some claim that air emboli are only an issue when introduced into arteries and that venous air emboli diffuse into the lungs when passing through the lung capillary bed, however many report adverse reactions when suspensions containing air bubbles are injected intravenously.
- It is probable that air-bubbles in the Clodrosome® suspension are stabilized by a monolayer of lipid, and therefore may not be as readily dissolved as air bubbles in an aqueous solution. This is characteristic of liposome suspensions and does not indicate a quality issue with Clodrosome®. However, if a substantial increase in bubbles or foam is noticed with older Clodrosome® suspensions, it may be an indication of degraded lipid and the Clodrosome® should not be used.
- The other important reason for removing all air bubbles is that included air bubbles occupy space in the needle and syringe so that the volume of suspension in the needle/syringe is less than the measured volume.
- Syringes are calibrated to deliver the indicated volume with the syringe tip and needle hub completely filled.
- It is not an issue when dosing Clodrosome® directly from the vial, but the dead volume in a 1 ml syringe and needle hub is substantial (70 µl) compared to the 100-200 µl dose intended for mice. If this dead volume is not taken into account when using a single 1 ml syringe to draw up and/or dilute a suspension within the syringe, significant dosing errors can occur [10].
- If necessary, a temporary engorgement of the vein may make the injection easier.
- For example, some technicians briefly dip the tail into warm—not HOT—water in order to make the vein more prominent; warm compresses may be applied to rabbit ears; tourniquets are appropriate for use on limbs in dogs.
- Some technicians place animals under heat lamps, but this is discouraged, especially in small rodents, as the animals are often overheated leading to additional trauma and discomfort.
- If the vein is not easily visualized or accessed, consider using another injection route, such as retro-orbital injection as described above.
- Remember that any change in protocol usually must be approved by the institutional animal care and use committee, therefore it is often useful to include alternative injection sites and/or methods in the initial application to the committee.
- Once the needle is inserted into the tail vein, slowly and steadily inject the suspension at a rate of no more than 1 ml/minute. This translates to taking about 12-15 seconds to inject a 0.2 ml dose volume. Should the animal become overly agitated or begin gasping for air as discussed above, further decrease the injection rate. The slow injection rate allows the liposome suspension to be thoroughly mixed into the blood so that they are properly opsonized for optimal phagocyte uptake.
- The animals should be closely observed for a few minutes for adverse reactions after the injection is completed and the animal returned to the cage. The investigator should consider excluding animals from the study which demonstrate significant aberrant behavior.
Margination of Circulating Monocytes
The entire population of monocytes (or neutrophils) within the vasculature at any given time is not represented in a blood sample. Margination is the term used to describe the adhesion of monocytes, or other cells, to the vascular endothelium; margination occurs under both steady state and inflammatory conditions and precedes the extravasation of monocytes into the tissue where they mature into tissue macrophages or dendritic cells. A review of the mechanism and cell surface molecules involved in margination was published by Beekhuizen and van Furth [21]. Ohgami, et al. reported that 5-7X more monocytes lined the vasculature of the lungs in rabbits than were found in the circulation while the number of marginated neutrophils was 2-3X that of the circulating neutrophil population [22]. These authors and others speculate that the margination is in preparation for replacing depleted alveolar macrophages. van Furth and Sluiter had previously reported a 60-40 distribution of marginated-circulating monocyte population in Swiss mice based on tail-vein blood collection rather than lungs and further speculate that the marginated and circulating monocytes exchange readily as has been shown for neutrophils [23]. Although their focus was on the mechanism of granulocyte margination, Fehr and Jacob had already identified complement activation as at least one factor which induced margination of both monocytes and granulocytes in rabbits as well as humans receiving dialysis [24]. Given that liposomes are known to activate complement [25, 26], it is not surprising that Sunderkötter, et al. showed that DiD-labeled liposomes (fluorescent clodronate liposome controls with no encapsulated clodronate) induced the margination of monocytes in mice which began to resolve somewhere between 4 and 16 hours post-injection [27]. However, these authors attribute the margination phenomenon to a cellular reaction upon phagocytosis of liposomes. Although the fact that the monocyte margination was more likely due to complement activation rather than phagocytotic events, we do not believe that the authors other conclusions would have been disputed by this realization. What is most important when reviewing the Sunderkötter, et al. results is that apparent monocyte “depletion” at early time points (at least before 4 hours, but probably even longer) can be attributed to margination rather than actual monocyte death. Furthermore, as early as 2 hours post-liposome injection, new monocytes from the bone marrow began to appear in the bloodstream in these animals but not in clodronate liposome-treated mice, suggesting that the clodronate liposomes also depleted the immature monocytes in the bone marrow since clodronate liposomes would not have remained in the circulation for more than 2 hours. However, immature monocytes (Ly-6C hi ) began to reappear in the circulation sometime between 24 and 30 hours and reach baseline counts before 48 hours, although the steady-state distribution of Ly-6C lo (mature) vs Ly-6C hi(immature) monocytes was not re-established until somewhere between 4 and 7 days. The authors further discuss the differences between these monocyte subpopulations which should be considered when looking at circulating monocyte behavior before and after depletion.
Monocyte margination post-low-level-LPS challenge appeared to be responsible for acute lung injury according to O’Dea, et al. [29] and that the margination elicited monocyte egress from the bone marrow. Lung injury correlated to the newly circulating monocytes preferentially marginating to the lung. Monocytes marginate to other organs in addition to the lungs during LPS-challenge and may contribute to sepsis-induced damage to these organs as well. It is not clear to us whether or not marginated macrophages phagocytose clodronate liposomes in normal animals, but Bouwens and Wisse reported marginated monocyte uptake of latex particles in a model in which margination of monocytes in the liver was accomplished by Zymosan-(particles prepared from yeast membranes)induced inflammation [30]. Therefore, we might assume that marginated monocytes will be depleted in models where inflammation exists at the time of clodronate liposome administration. Clearly, the status and contribution of marginated monocytes requires additional investigation. Some report that any increased stress in such as restraint during the act of collecting a blood sample can affect the number of circulating monocytes.
RES blockade is the result of the saturation of blood-accessible phagocytic cells such that circulating liposomes or other particles are not removed from the bloodstream [32]. There is also evidence that depletion of blood borne opsonins may contribute to the hampered particle uptake [26]. Liposomes, latex beads, Zymosan are examples of particles which effectively induce RES blockade. Phagocytes can digest liposomes within a day so that the blockade is reversible, however latex cannot be metabolized and the phagocyte seems to retain some level of impairment until it is replaced after its normal lifespan. Therefore, it does make a difference as to which agent is used to accomplish the blockade. The application of RES blockade is discussed in detail on the Mannosylated Liposomes page under A Strategy for Targeting Phagocytes Resistant to Clodronate Liposome Depletion. RES blockade may be induced by dosing the particles either through the vascular or the peritoneal route, however vascular dosing is somewhat more efficient.
Rates of Macrophage Repopulation
The steady state distribution of macrophage subpopulations may not be represented during repopulation post-depletion as discussed earlier for monocytes. Therefore, if a study involves the behavior of a phagocyte class during the repopulation phase, those results may not be reflective of the steady state behavior of those cells. However, the most confounding issue for many is the fact that monocyte repopulation may begin as early as 24 hours while spleen and liver macrophage depletion can last as long as 7 days. Therefore, if complete systemic macrophage depletion or just monocyte depletion is the goal, daily injections of clodronate liposomes may be necessary for experimental protocols requiring data collection more than 1 day post injection of the clodronate liposomes. Also take into account that the results on monocyte repopulation reported above were obtained in mice and other species may demonstrate different repopulation kinetics as has been shown for splenic macrophage repopulation between mice and rats.
In conclusion, intravenous administration is the most commonly used method for dosing clodronate liposomes, however many factors still need to be taken into account to ensure that the depletion provides the required results. Phagocyte depletion should always be verified in the tissue(s) of interest and results cannot be extrapolated from species to species. Even amongst mice of the same strain, knockouts or other genetically altered animals may have resulting traits which could alter their response to clodronate liposomes. Thus, careful consideration of the effect of clodronate liposomes through a thorough understanding of their expected behavior post-intravenous injection must accompany every study. Developing the skills for designing the appropriate experiments and controls to explain unexpected results as well as achieve the desired results must also be integrated into the use of clodronate liposomes for phagocyte depletion.
References
18. Lin JH. Bisphosphonates: a review of their pharmacokinetic properties. Bone. 1996 Feb 1;18(2):75-85.
23. Van Furth R, Sluiter W. Distribution of blood monocytes between a marginating and a circulating pool. Journal of Experimental Medicine. 1986 Feb 1;163(2):474-9Van Furth R, Sluiter W. Distribution of blood monocytes between a marginating and a circulating pool. Journal of Experimental Medicine. 1986 Feb 1;163(2):474-9.